Featured
Reprocessed GRACE-FO density and crosswind data available on Swarm dissemination Server
New Invitation To Tender - Data Fusion Toolbox for Regional High-Latitude Ionospheric Electrodynamics
Swarm Alpha and Bravo EFI Gain Map calibration and partial data gap on 25 June 2024
Swarm L1B ORBATT and MAGNET operational processor updated
About Swarm
Swarm is ESA's first constellation mission for Earth Observation (EO). The mission consists of three identical satellites named Alpha, Bravo, and Charlie (A, B and C); which were launched on 22 November 2013 into a near-polar orbit.
Swarm is dedicated to creating a highly detailed survey of Earth's geomagnetic field and its temporal evolution as well as the electric field in the atmosphere, by using a satellite constellation that carries sophisticated magnetometers and other instruments.
The initial constellation of the mission was achieved on 17 April 2014. Swarm A and C form the lower pair of satellites flying side-by-side (1.4° separation in longitude at the equator) at an altitude of 462 km (initial altitude) and at 87.35° inclination angle, whereas Swarm B is cruising at a higher orbit of 511 km (initial altitude) and at 87.75° inclination angle. Due to the subtle difference in altitude and inclination, the Swarm B orbit drifts slowly away from the Swarm A/C orbits at a rate of about 24°/year (difference in longitude of the ascending node). In October 2021, the orbital planes realigned, Swarm B counter-rotating with respect to Swarm A and C. To maximise the scientific return of this conjunction, the Swarm A and C satellites were brought back into the same orbital plane, starting with a manoeuvre in October 2019 that would slowly reduce the separation in longitude, achieving a perfect alignment at the exact time of the conjunction with Swarm B. During the conjunction, the distance between the Swarm A and C satellites was reduced to only 2 s along the orbit (15 km), which was previously kept between 4–10 s for safety reasons.
In March 2018, the CASSIOPE/e-POP mission was formally integrated into the Swarm constellation as the fourth element (Swarm-E) under ESA's Earthnet Third Party Mission Programme. The e-POP payload operations are currently focused on maximising the Swarm/e-POP scientific outcome, and there is an ongoing joint effort to develop new and better-calibrated products based on e-POP data.
Swarm was designed to operate for four years, following a three-month commissioning phase. In November 2017, the mission was granted a four-year extension to 2021. It was then extended further and will operate till 2025.
Swarm Objectives
The Swarm mission primary objectives are:
- Study of core dynamics, geodynamo processes and core-mantle interaction
- Mapping of lithospheric magnetisation and its geological interpretation
- Determination of the 3D electrical conductivity of the mantle
- Investigatigation of electric currents flowing in the magnetosphere and ionosphere.
The secondary objectives are:
- Identifying the ocean circulation by its magnetic signature
- Quantifying the magnetic forcing of the upper atmosphere.
Swarm Instruments
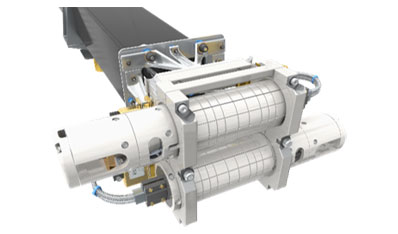
find out more
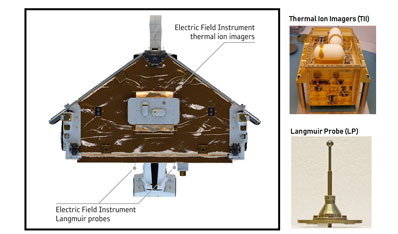
find out more
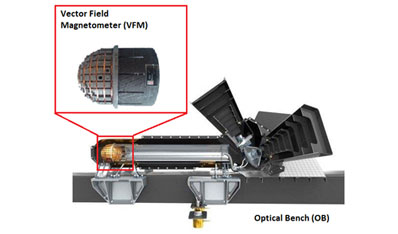
find out more

find out more
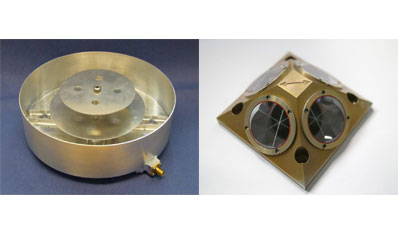
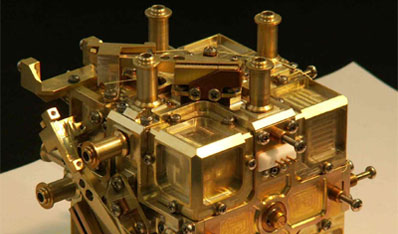
find out more